Background
The concept of a nuclear space reactor is not new. The U.S. developed the first space reactor in 1965 for propulsion of the SNAP 10A experimental satellite (pictured below). Between 1967 and 1988, the Soviet Union built 35 space reactors to power satellites. All 36 reactors were fueled with highly enriched uranium (HEU), which is the same material used to make nuclear bombs. But since 1988, neither the U.S. nor Russia has launched a space reactor. (Note: some have suggested that space reactor launches were halted because major accidents occurred in over 20% of space reactor missions.) [1]
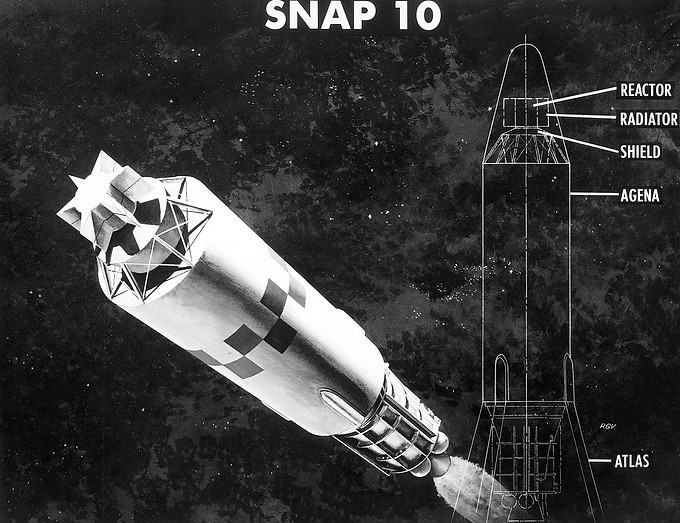
Source: US Department of Energy (https://www.flickr.com/photos/departmentofenergy/10950455806/in/photostream/)
In the intervening years between 1988 and the present, the U.S. has expanded its commitment to minimizing the commercial use of HEU, hoping to prevent diversion or theft of the sensitive material for proliferation or terrorism. The commitment reflects an understanding that HEU is one of the most dangerous substances on earth. The sensitive material can easily be used to create a nuclear explosion. The simplest nuclear bomb design, known as the gun-type, uses HEU. A gun-type bomb slams two subcritical quantities of HEU together to create an explosion. The nuclear bomb dropped on Hiroshima used a gun-type design. A single individual could even create a nuclear blast by simply dropping a 25 kg. piece of HEU onto another 25 kg. piece of HEU.
​
However, the U.S. did not always recognize the dangers of HEU. Only five years after dropping two atom bombs on Japan, the U.S. began exporting HEU as fuel for research reactors. In the 1950s, the U.S. and Soviet Union supplied more than 40 countries with research reactors. [2] These reactors were originally meant to burn low-enriched uranium (LEU), but after some “technical difficulties,” the U.S. decided to supply states with weapons-grade fuel instead. [3] The U.S. and Soviet Union also developed HEU-fueled reactors for naval propulsion, and, as noted above, both superpowers launched space reactors fueled with weapons-grade HEU. [4]
​
These three non-weapons uses of HEU contributed greatly to the global spread of HEU in the 1950s-1970s. (Note: other civilian applications of HEU also contributed to this growth, including medical isotope production, critical assemblies, and pulsed reactors.) U.S. exports alone accounted for around 3 tons of HEU traded annually by the mid-1960s. This is disturbing considering that only 25 kg. of HEU, or less, is needed to make an implosion weapon. [5]
​
India’s successful nuclear bomb test in 1974 revealed how easily states could repurpose civilian nuclear material and technology to proliferate. In response, the U.S. reversed course and began leading efforts to minimize global HEU commerce. [6] In 1978, U.S. officials started the Reduced Enrichment for Research and Test Reactors (RERTR) program, which was later incorporated under the U.S. Global Threat Reduction Initiative (GTRI). The program worked directly with “operators of nuclear facilities” across the world to convert research reactors from HEU to LEU. [7] By 2018, around 100 research reactors had been converted or shut down. [8]
​
The Soviet Union launched a program similar to RERTR in the 1970s. The Soviet Union aimed to convert foreign reactors that it supplied “from 80% to 36% enriched fuel.” [9] (Note: the technical definition of LEU is less than 20% enriched.) The program faltered in the early 1990s, but U.S. assistance revived Russian efforts by 1993, this time aimed at converting to LEU.
​
With the end of the Cold War, the U.S. also worked with Russia to reduce Russia's HEU stocks. The Megatons to Megawatts agreement, signed in 1993 and completed in 2014, required Russia to downblend 500 tons of HEU from dismantled nuclear weapons to LEU. [10] The U.S. then purchased the LEU for energy production. In 1999, the U.S. developed the Materials Consolidation and Conversion (MCC) program as the civilian counterpart to Megatons to Megawatts. Through MCC, the U.S. purchased excess Russian civilian HEU for downblending to LEU. [11]
​
In addition, the U.S. worked to reduce the stockpiles of HEU that foreign reactors had already used. When reactors burn HEU, they produce spent fuel that contains “about half” of the U-235 found in the original HEU, meaning that the spent fuel still holds “near weapons grade” uranium. [12] In 1968, under its Off-Site Fuel Policy, the U.S. began to take back spent fuel produced from U.S.-origin HEU. The Off-Site Fuel Policy was stopped in the late 1980s, but in 1996, the U.S again started to take back spent fuel from American HEU. [13] The U.S. also cooperated with Russia to develop a parallel mechanism. Thus, in 2002, Russia started retrieving “Soviet/Russian-origin HEU fuel from outside Russia.” [14]
​
Understanding the risks associated with HEU, the U.S. Congress even tried to phase out all U.S. HEU exports. In 1992, Congress passed the Schumer Amendment to the Energy Policy Act. The Amendment allowed the export of HEU only if three criteria were met: (1) the foreign facility was unable to fuel its reactor with any “existing alternative LEU fuel,” (2) the foreign facility agreed to convert to LEU as soon as possible, and (3) the U.S. actively developed “an alternative LEU fuel suitable for that facility.” [15] Four years after the Amendment’s enactment, all HEU exports stopped temporarily. [16] In 2005, passage of the Burr Amendment walked back some of the Schumer restrictions for the production of medical isotopes.
​
In 2009, President Obama announced that the US intended to “secure all vulnerable nuclear materials around the world within four years.” [17] The decision led to the Nuclear Security Summits. The first Summit Work Plan asked its members to limit the use of HEU in civilian applications. By 2016, the final Summit had produced a Joint Statement that committed its 21 signatories, including the US, to “make every effort to achieve further progress with regard to minimizing and eliminating the use of HEU in civilian applications.” [18] The document formalized the US commitment to HEU minimization, but notably did not discuss space reactor technology.
​
The U.S. efforts discussed above have contributed to a decline in HEU commerce. Certain non-weapons uses still consume large quantities of the material (primarily naval propulsion), but the U.S. has spearheaded the global move away from HEU. The fact that NASA plans to use HEU to fuel its space reactor therefore threatens to undermine the last 40 years of U.S. leadership on HEU minimization.
In January 2018, NASA tested a nuclear reactor for use in space. The proposed reactor (shown in NASA’s promotional video) would power an enduring installation on a planetary surface such as the Moon or Mars. NASA envisions the planetary surface reactor, also known as Kilopower, as supporting President Trump’s desire to achieve “a sustainable human presence on the Moon by 2028.” [19] NASA tested the Kilopower reactor with HEU fuel.
​
NASA has turned to HEU because “LEU fuel typically requires more mass to produce the same energy as HEU fuel,” and because NASA cannot feasibly develop a LEU alternative in time to meet the 2028 deadline. [20] But these two constraints provide weak justification for HEU use. As noted by Dr. Jeffrey C. King in a 2019 symposium on space reactors, NASA could develop moderated reactors that use LEU while only marginally increasing system mass. [21] Even the Department of Energy concluded after a six-month reactor conceptual design study that moderated reactors fueled with High-Assay Low Enriched Uranium (uranium that is enriched between 5% and 20%) “are competitive in mass with HEU-based designs but have greater complexity.” [22] The missions planned by NASA in the near future can likely use LEU without exceeding weight constraints. Also, Congress has already signaled that it may delay President Trump’s timeline for a lunar mission. NASA could use the lag in Congressional authorization to develop an alternative LEU reactor, as advised by Representative Bill Foster, whose amendment passed in a FY2020 appropriations bill, instructing NASA to “work towards the development of a LEU space power reactor.” [23] The Senate Commerce Committee passed a similar amendment sponsored by Senator Edward Markey that instructed the "prioritization of low-enriched uranium technology" for space technology." [24]
​
NASA’s decision to use HEU is even more surprising in light of the associated costs, such as far greater security. HEU, as a DOE Category 1 Material, requires more protection from theft and diversion than LEU. NASA could offset some of these security costs by using DOE facilities, but at launch sites, NASA will have to pay full price. NASA’s Nuclear Power Assessment Study estimates that the security upgrades needed to handle HEU will cost around $70 million for a first launch. [25] NASA will also have to account for the risk of losing HEU in a launch failure. At minimum, this risk would require NASA to pay for “an emergency search team to retrieve HEU in case of launch failure.” [26]
​
NASA will also have to pay greater research, design, and production costs due to the lack of commercial partners licensed to handle HEU. NASA could save money by collaborating with private companies that have already developed commercial reactors, but all but one of these companies lack the licenses necessary to work with HEU. Thus, NASA’s use of HEU will prevent cost-saving partnership by excluding “private industry from taking part in space-reactor research and development.” [27]
​
The greatest flaw in NASA’s plan, however, is that it overlooks the cost of reneging on the U.S.-led campaign to limit civilian use of HEU. The U.S. has helped establish an international norm against non-weapons uses of HEU to facilitate these minimization efforts. If the U.S. uses HEU in space reactors, then it will “undermine the norm against civil HEU use” and will provide a justification for other countries to use HEU in their applications. [28]
​
Consider the U.S. attempt to build a new HEU-fueled reactor in the late 1980s. DOE announced that it planned to use HEU in the Advanced Neutron Source reactor. The decision cast doubt on America’s commitment to ongoing minimization efforts, namely the RERTR program. Countries responded by accusing the U.S. of applying a double standard. French officials threatened that they would “insist on using HEU in their best research reactors” if the U.S. proceeded to build the reactor. [29] In Germany, operators responded to the U.S. hypocrisy by actually building an HEU-fueled research reactor. [30]
​
This case demonstrates that the U.S. cannot impose restrictions on the international community while holding itself to a separate standard. NASA’s Kilopower reactor will provide justification for other national space programs to fuel their space reactors with HEU. If countries begin racing to develop HEU-fueled space reactors, the demand for HEU will quickly surpass the supply in many countries. That would then incentivize states to establish new HEU enrichment facilities, further undermining the minimization norm.
​
Finally, because NASA’s plan undermines U.S. minimization leadership, it will incite political opposition to space reactors from the nonproliferation community. The community protested the construction of the proposed Advanced Neutron Source reactor discussed above. Nonproliferation advocates successfully convinced President Bill Clinton, who cancelled the project in 1995, that the HEU-fueled reactor constituted an “important non-proliferation policy concern.” [31] If the community can convince policymakers again that civilian HEU use threatens nonproliferation, then NASA will face a significant hurdle to the development of Kilopower.
​
​
​
​
​
[1] Christopher Fichtlscherer, “Do we need highly enriched uranium in space (again)?” Bulletin of Atomic Scientists, September 12, 2019, https://thebulletin.org/2019/09/do-we-need-highly-enriched-uranium-in-space-again/.
[2] Alexander Glaser and Frank N. Von Hippel, "Global Cleanout: Reducing the Threat of HEU-Fueled Nuclear Terrorism," Arms Control Today (2006): 19.
[3] Alan J. Kuperman, “Nuclear Nonproliferation via Coercion and Consensus: The Success and Limits of the RERTR Program (1978–2004),” in International Cooperation on WMD Nonproliferation, ed. Jeffrey W. Knopf (Athens, GA: University of Georgia Press, 2016): 47.
[4] Alan J. Kuperman, “Global HEU Phase-Out: Prospects & Challenges,” in Nuclear Terrorism and Global Security: The Challenge of Phasing out Highly Enriched Uranium, ed. Alan J. Kuperman (New York: Routledge, 2013): 4.
[5] Kuperman, "Global HEU Phase-Out," 5.
[6] Kuperman, "Global HEU Phase-Out," 6.
[7] Ibid., 7.
[8] Edwin Lyman. “The Space Reactor Community Must Preserve the Norm Against the Civil Use of HEU.” Panel presentation, Nuclear Energy in Space: Nonproliferation Risks and Solutions, Washington D.C., October 17, 2019. http://sites.utexas.edu/nppp/space-symposium/.
[9] Glaser and Von Hippel, "Global Cleanout," 19.
[10] Corey Hinderstein, Andrew Newman, and Ole Reistad, “From HEU Minimization to Elimination: Time to Change the Vocabulary,” Bulletin of the Atomic Scientists 68, no. 4 (2012): 85.
[11] Glaser and Von Hippel, "Global Cleanout," 21.
[12] Ibid., 21.
[13] Alexander Glaser and Frank N. Von Hippel, “Thwarting Nuclear Terrorism,” Scientific American 294, no. 2 (2006): 60.
[14] Glaser and Von Hippel, "Global Cleanout," 21.
[15] “Civilian HEU: United States,” Nuclear Threat Initiative, last modified June 28, 2019, https://www.nti.org/analysis/articles/civilian-heu-united-states/.
[16] "Civilian HEU."
[17] “Past and Current Civilian HEU Reduction Efforts,” Nuclear Threat Initiative, last modified December 20, 2017, https://www.nti.org/analysis/articles/past-and-current-civilian-heu-reduction-efforts/
[18]"Joint Statement on Minimizing and Eliminating the Use of Highly Enriched Uranium in Civilian Applications," IAEA Information Circular, February 16, 2017. https://www.iaea.org/sites/default/files/publications/documents/infcircs/2017/infcirc912.pdf.
[19] Alan J. Kuperman, “Rapporteur’s Summary,” Nuclear Energy in Space: Nonproliferation Risks and Solutions, Washington D.C., October 17, 2019. http://sites.utexas.edu/nppp/space-symposium/.
[20] Blake Messer, “Space Reactors,” in in Nuclear Terrorism and Global Security: The Challenge of Phasing out Highly Enriched Uranium, ed. Alan J. Kuperman (New York: Routledge, 2013): 212.
[21] Jeffrey C. King, “Space Nuclear Power Systems – LEU vs HEU Tradeoffs,” Panel presentation, Nuclear Energy in Space: Nonproliferation Risks and Solutions, Washington D.C., October 17, 2019. http://sites.utexas.edu/nppp/space-symposium/.
[22] Lee Mason, “NASA STMD Nuclear Technology Portfolio,” Panel presentation, American Nuclear Society Annual Meeting, online virtual meeting, June 11, 2020.
[23] Bill Foster, “Space reactors and proliferation,” Keynote presentation, Nuclear Energy in Space: Nonproliferation Risks and Solutions, Washington D.C., October 17, 2019. http://sites.utexas.edu/nppp/space-symposium/.
[24] Jeff Foust, "Senate committee approves NASA authorization bill," Space News, November 13, 2019. https://spacenews.com/senate-committee-approves-nasa-authorization-bill-2/
[25] Ralph L. McNutt and Paul H. Ostdiek, “Nuclear Power Assessment Study Final Report,” prepared by The Johns Hopkins University Applied Physics Laboratory, Laurel, February 4, 2015. file:///Users/connerjoyce/Downloads/NPAS_Final_Report%20(1).pdf.
[26] Kuperman, “Rapporteur’s Summary.”
[27] Fichtlscherer, "Do we need HEU"
[28] Lyman, "The Space Reactor Community."
[29] Kuperman, "The Success and Limits of RERTR," 58.
[30] Ibid., 58.
[31] Alan J. Kuperman, “The US Navy Should Start Weaning its Reactors off Bomb-Grade Uranium,” Defense One, March 13, 2018.
​